Biomechanics Mysterious muscle forces
Where does the strength of stretched muscles come from?
Sitting down while carrying a heavy backpack can be easily accomplished. But how do you get back up? Most of the time not without help. To biomechanists like Prof Dr Daniel Hahn and his team this everyday phenomenon poses quite a conundrum. It cannot be explained by the established theories on muscle.
The secret behind the different muscle forces lies in the stretch of muscle. Many movements, such as walking, are based on muscles being repeatedly stretched, before actively contracting. “If I lift a weight, the respective muscle shortens, which is called a concentric contraction,” explains Daniel Hahn. “But if I succumb to gravity, because I sit down or walk downstairs, the muscle is stretched. Nevertheless, it is active, because it has to control the movement. We refer to this as eccentric contractions.”
More power – less energy
Experiments have shown that the strength generated by muscle during eccentric contraction is much greater than the strength generated during concentric contraction. In the process, less energy is consumed. “The reason for this phenomenon is highly debated,” says the sports scientist.
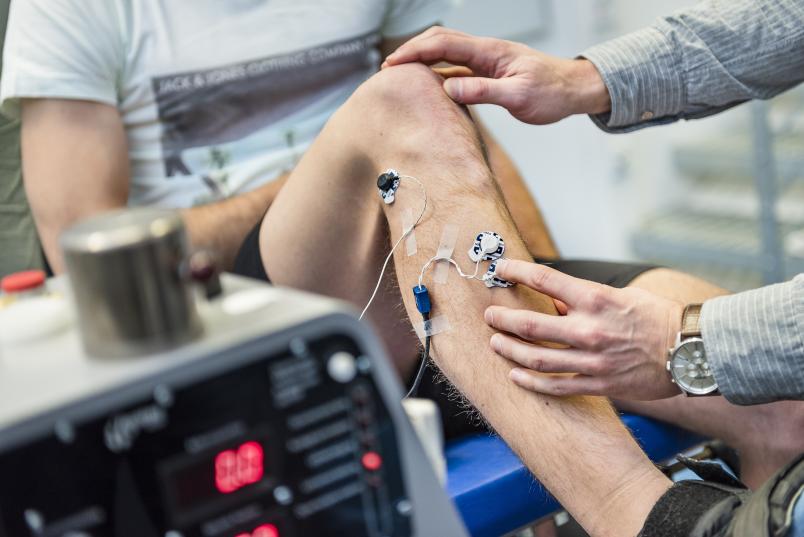
In order to find out what causes that difference, researchers initially examined isolated muscles. For this purpose, individual muscle cells, for example those of rats, are artificially activated while remaining at constant length or being stretched. Under these conditions it emerged that the strength of a stretched muscle is 1.5 to 2.5 times greater compared to that of a muscle that is not stretched.
Tests with participants who were asked to pull a lever while a machine was pulling the same lever back, thus stretching their muscle, yielded a different result, for the most part: here, the strength during eccentric contractions was hardly any greater or not greater at all than the strength during a contraction where the muscle was not stretched.
Ultrasound makes muscle fibres visible
As these results contradicted not only muscle experiments, but also everyday experience with eccentric contractions, they aroused the researchers’ interest. “When we talk about a muscle in humans, we actually refer to a complex that also includes tendons, connective tissue, and the nervous system that controls the muscle,” describes Daniel Hahn. In order to unravel the secrets of eccentric strength during stretching in humans, each of these components has to be studied.
“Increased muscular strength can occur only if the muscle fibres are stretched,” explains Daniel Hahn. “If I bend my knee while walking downstairs, elastic tendons can buffer the stretch of the knee extensor muscles so that the muscle fibres are not stretched at all." This would explain why no increased eccentric strength was identified in many of the experiments with human participants.
In order to ensure in their own experiments that the muscle fibres were stretched, Hahn and his team at the Department of Human Movement Science used B-mode ultrasound. As ultrasound renders muscle fascicles visible during contraction, it is possible to check if they are actually stretched.
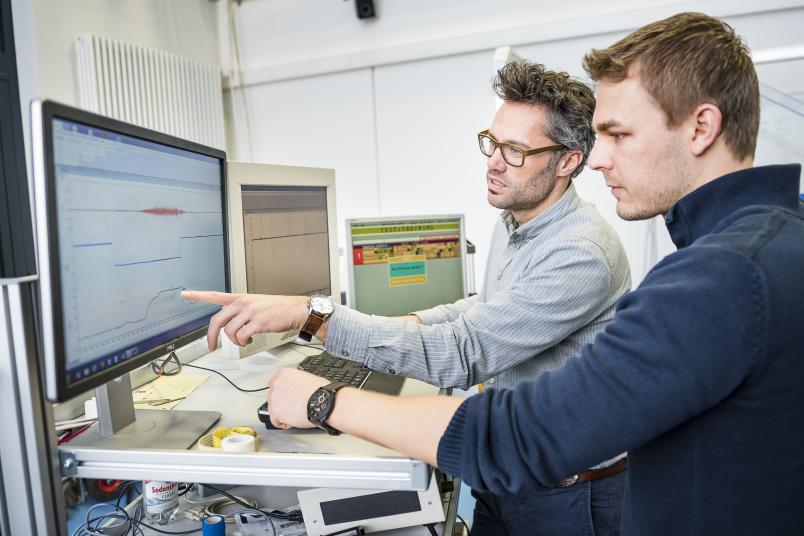
Using this method, the researchers successfully verified that the test participants produced between 1.2 and 1.4 times as much strength during eccentric contractions compared to contractions with no stretch. And more than that: Even after the stretch was stopped, the strength remained approximately ten percent higher as long as the participants continued to activate their muscle.
Additionally, the researchers in Hahn’s team scrutinised the neuronal aspects of muscle contraction, namely the control of muscle activity that starts in the brain and proceeds via the spinal cord and the respective nerve pathways. The signals always travel in both directions: motor signals from the brain to the muscle, sensory signals from the muscle to the brain. The researchers analysed those signals and their effects in a number of experiments.
Stimulation with a magnetic coil through the skull
They measured the muscle activity when the muscle was stretched and kept at constant length – not only when the participants activated their muscles voluntarily, but also when contractions were elicited through electric stimulation applied to either the nerve or the spinal cord, or through transcranial magnetic stimulation. For this purpose, brain regions of the motor cortex that are responsible for activating specific muscles were stimulated with a magnetic coil through the skull.
As the increase of muscle strength during eccentric contractions in living organisms is less than that in isolated muscle cells, it appeared that the strength was inhibited by the nervous system. “But we weren’t able to prove this hypothesis in our experiments,” points out Daniel Hahn.
What the researchers were able to prove was the fact that the nerve cells in the brain were more excitable after the muscle had been stretched, while the participants maintained the tension, than when no stretching had occurred. This phenomenon might be one of potentially several factors that contribute to an increase in strength after stretching. The reason for the increased excitability hasn’t yet been fully understood.
In terms of the muscle itself, Hahn and his colleagues suspect that there might be another reason for the increase in strength of stretched muscles: a protein called titin.
Prior to that, it had been overlooked. Interestingly enough not because of its small size, but because of its large size.
Daniel Hahn
Muscle contractions are caused by two overlapping proteins in the muscle cells – a thin filament composed of the protein actin and a thick filament composed of the protein myosin – sliding into one another. The muscle thus shortens. This process was first described by the so-called sliding filament and cross-bridge theories in 1954 and 1957.
It wasn’t until 20 years later that a third muscle filament was discovered: namely titin. “Prior to that, it had been overlooked. Interestingly enough not because of its small size, but because of its large size,” says Hahn. Titin might fulfil the function of a molecular spring that counteracts or assists contractions in the muscle cell. Experiments have shown that this spring changes its properties dynamically – i.e. it gets stiffer when the muscle is stretched. As a result, less energy input would be needed to generate greater forces during stretch of muscle cells.
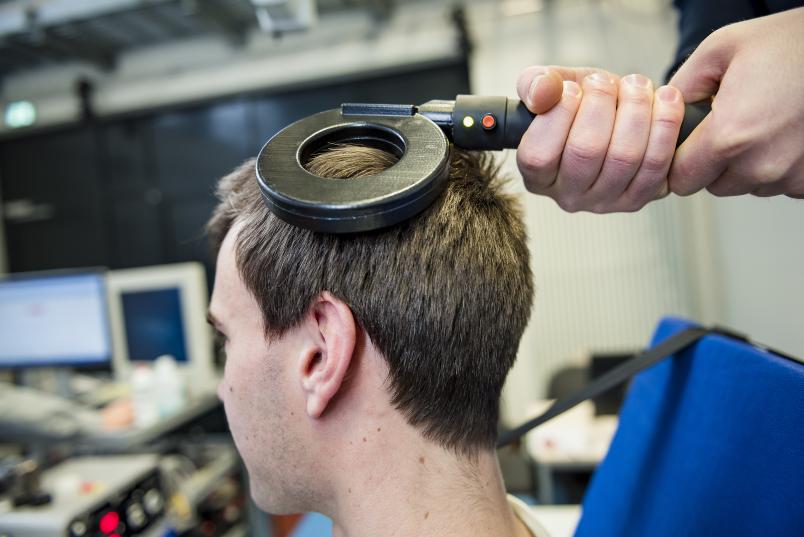
The research group from Bochum will continue to explore these questions in the course of a new project funded by the German Research Foundation in 2018. The project will focus on investigating to what extent the nervous systems, tendons and muscles affect the amplification of force and power production during and after eccentric contractions.
“The results are relevant not only in the field of basic research or performance enhancement in sports, but also for prosthetic and robotic technology,” says Daniel Hahn. Studies conducted by US-American researchers have demonstrated that an exoskeleton where the leg is supported by an external spring resulted in energy conservation of seven percent during walking.
Prosthetics could thus be moved in a more natural manner and facilitate a smooth gait.
Daniel Hahn
Once the neuromuscular mechanisms are better understood, such supporting systems might feasibly become more and more sophisticated and adjusted to natural movements – for example walking. A spring that changes its properties, for example by getting stiffer once the heel makes contact with the ground and then returning to a compliant state, might help weakened or injured individuals maintain their independence and mobility.
“The more we learn about the details of complex motions, the better our ability to build artificial systems that may replace body parts,” as Hahn predicts for the future. “Prosthetics could thus be moved in a more natural manner and facilitate a smooth gait.”