Computational Mechanics
Should vasoconstriction be treated or not?
Engineering teams simulate the behaviour of blood vessels. This is done to assess whether treatment is necessary and what it should look like.
If deposits have formed in arteries that threaten to block the blood vessel or become loose and might block smaller vessels, doctors are faced with the question of whether and how they should intervene. One possibility to widen a narrowed vessel is balloon dilatation. In this procedure, a small balloon is pushed from the patient’s groin through a catheter to the calcified area of the artery, where it is inflated with pressure. This causes the vessel to dilate, and the blood can flow again.
However, this procedure can also go wrong. If the pressure in the balloon is too high, the blood vessel is dilated too much and the plaque can tear. Or the damage to the vessel wall can be so severe that complications arise at a later point.
How high is the probability of complications
In order to predict and avoid such incidents, researchers at the Department of Civil Engineering make complex calculations. Mechanical engineers Professor Daniel Balzani and Professor Klaus Hackl researched into simulating the behaviour of elastic tissue such as blood vessels. In the process, Daniel Balzani and his team focus on calculating the risk of possible damage in advance. “Based on ultrasound examinations of the vessel, a doctor will ideally be able to determine the probability that a narrowed spot in the vessel might cause a stroke or heart attack, for example,” explains Daniel Balzani. “This enables him to make more informed therapeutic decisions and avoid unnecessary interventions and thus complications. It also saves financial resources, which can then be used more effectively.”
However, calculating such a risk is anything but simple. Basically, knowledge about the material properties of blood vessels can be gained from animal experiments or by analysing the vessels of deceased persons. “We therefore work with simplified and automated models, in which we integrate as many vessel properties as possible to enable patient-specific simulations,” elaborates Daniel Balzani.
A deposit like this is not firm in general. It may feel like wet beach sand.
Daniel Balzani
The fibres inside the vessel run transversely to the blood flow, whereas further outside they run diagonally. In addition to the orientation of fibres, many other effects must be taken into account in simulations, including the elasticity of the vessel wall, residual stresses in the artery, the activity of the smooth muscle cells that surround the vessel and actively influence its diameter, and the damage caused by possible overstretching of the vessel. Not to mention the properties of the plaques that are responsible for the narrowing of blood vessels. “A deposit like this, though stiff, is not firm in general. It may feel like wet beach sand,” describes Daniel Balzani. Mechanical experiments are therefore difficult.
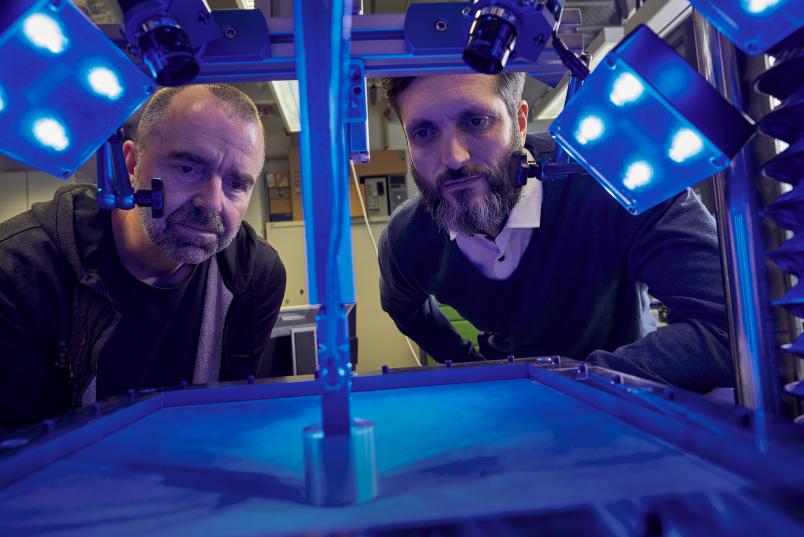
The engineers base their calculations on the fact that the structures develop in accordance with the strain put on them, much like muscles thicken when they are constantly exerted. “This information enables us to, so to speak, grow the optimal material in the simulation,” explains Balzani. The engineers develop a separate algorithm for each vascular property. They must, after all, be coupled and everything has to be calculated simultaneously. “We’re not quite there yet,” admits Daniel Balzani. Still, some combinations have already been implemented. Mainframe computers are required to calculate these interlocking algorithms. “But even those might take several days to simulate two heartbeats,” says Balzani.
Klaus Hackl and his team are also working on predicting the success of a possible balloon dilatation. But first and foremost, he focuses on the computer simulation of the healing of injured vessels. “Based on magnetic resonance or computer tomography of the affected vessel, we want to be able to tell the doctor where in a 3D scenario and with what pressure they should inflate the balloon best so that the treatment leads to the desired result and doesn’t cause such severe damage to the vessel wall that the artery can no longer heal,” says Hackl.
Vessels do not tear as if they were cut with a knife.
Klaus Hackl
He, too, struggles with complex material properties. “If we applied the rules of thermodynamics alone, our calculations for vascular injuries would show that, over time, the body would increasingly replace healthy tissue with diseased tissue,” he outlines. “However, this is something that the body can override, and we take it into account in our models.” The researchers compare the results of their calculations with experimental data from vessel studies, in order to verify whether the calculations yield plausible data. Hackl’s group also includes information about the elasticity of the arterial wall, as well as the properties of plaques and the balloon in the respective calculations. “For example, vessels do not tear as if they were cut with a knife, but along the fibres,” points out Hackl.
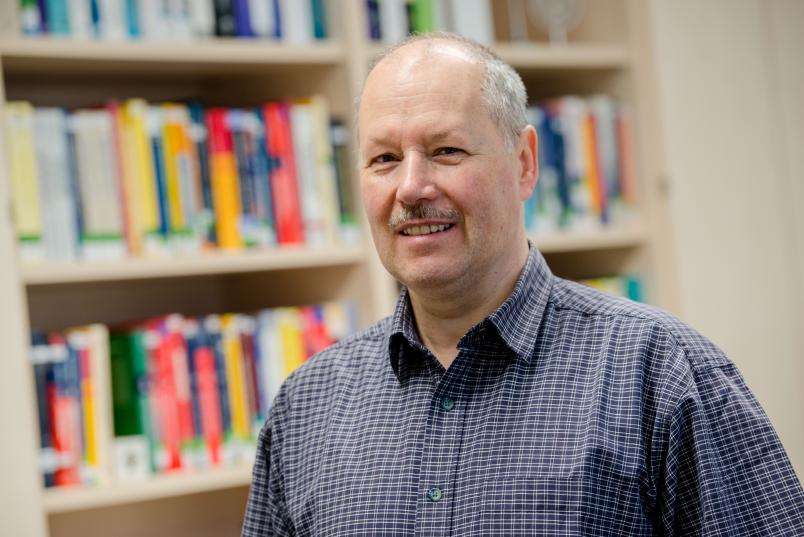
In addition to blood vessel injuries, he is also interested in the healing process of superficial wounds. In order to gain reliable prognoses, the researchers include a larger number of parameters pertaining to the properties of the skin and subdivide the wound into countless small fields. Everything is linked with everything and all parameters affect each other. “It will take a few years before we have a practical application,” Hackl estimates. “But in simple cases, such as the healing of different bones, the approach already works.”