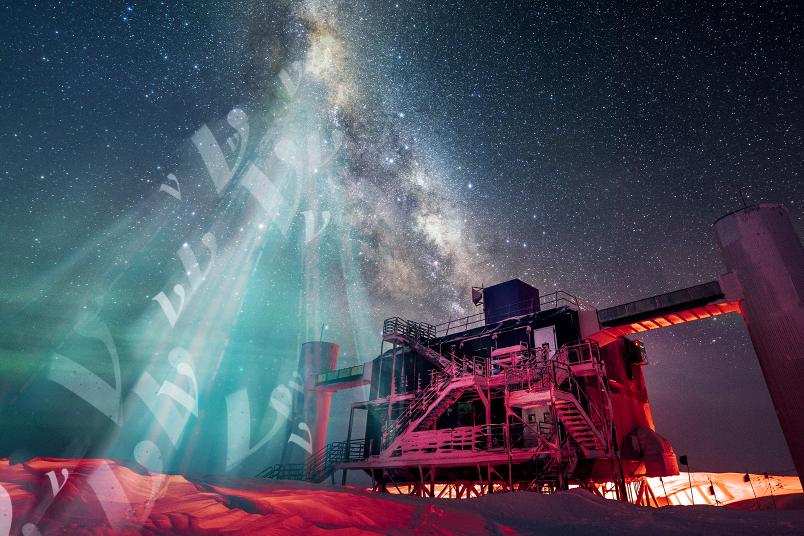
Astrophysics IceCube detector traces neutrinos from our Milky Way
The results enable us to see our galaxy through a new lens.
Our Milky Way galaxy is an awe-inspiring feature of the night sky, viewable with the naked eye as a horizon-to-horizon hazy band of stars. Now, for the first time, the IceCube Neutrino Observatory has produced an image of the Milky Way using neutrinos – tiny, ghostlike astronomical messengers. In an article published on 30 June 2023 in the journal Science, the IceCube Collaboration, an international group of over 350 scientists, presents evidence of high-energy neutrino emission from the Milky Way. The groups of the Bochum professors Julia Tjus and Anna Franckowiak contributed to this research.
A new image of the Milky Way
The high-energy neutrinos, with energies millions to billions of times higher than those produced by the fusion reactions that power stars, were detected by the IceCube Neutrino Observatory, a gigaton detector operating at the Amundsen-Scott South Pole Station. It was built and is operated with National Science Foundation (NSF) funding and additional support from the fourteen countries that host institutional members of the IceCube Collaboration. This one-of-a-kind detector encompasses a cubic kilometer of deep Antarctic ice instrumented with over 5,000 light sensors. IceCube searches for signs of high-energy neutrinos originating from our galaxy and beyond, out to the farthest reaches of the universe.
“What's intriguing is that, unlike the case for light of any wavelength, in neutrinos, the universe outshines the nearby sources in our own galaxy,“ says Francis Halzen, a professor of physics at the University of Wisconsin-Madison and principal investigator of IceCube. “As is so often the case, significant breakthroughs in science are enabled by advances in technology,“ says Denise Caldwell, director of NSF’s Physics Division. “The capabilities provided by the highly sensitive IceCube detector, coupled with new data analysis tools, have given us an entirely new view of our galaxy – one that had only been hinted at before. As these capabilities continue to be refined, we can look forward to watching this picture emerge with ever-increasing resolution, potentially revealing hidden features of our galaxy never before seen by humanity.“
Interactions between cosmic rays – high-energy protons and heavier nuclei, also produced in our galaxy – and galactic gas and dust inevitably produce both gamma rays and neutrinos. Given the observation of gamma rays from the galactic plane, the Milky Way was expected to be a source of high-energy neutrinos. “A neutrino counterpart has now been measured, thus confirming what we know about our galaxy and cosmic ray sources,” says Steve Sclafani, a physics PhD student at Drexel University, IceCube member, and co-lead analyzer.
The search focused on the southern sky, where the bulk of neutrino emission from the galactic plane is expected near the center of our galaxy. However, until now, the background of muons and neutrinos produced by cosmic-ray interactions with the Earth’s atmosphere posed significant challenges.
With the help of machine learning
To overcome them, IceCube collaborators at Drexel University developed analyses that select for cascade events, or neutrino interactions in the ice that result in roughly spherical showers of light. Because the deposited energy from cascade events starts within the instrumented volume, contamination of atmospheric muons and neutrinos is reduced. Ultimately, the higher purity of the cascade events gave a better sensitivity to astrophysical neutrinos from the southern sky.
However, the final breakthrough came from the implementation of machine learning methods, developed by IceCube collaborators at TU Dortmund University, that improve the identification of cascades produced by neutrinos as well as their direction and energy reconstruction. The observation of neutrinos from the Milky Way is a hallmark of the emerging critical value that machine learning provides in data analysis and event reconstruction in IceCube.
“The improved methods allowed us to retain over an order of magnitude more neutrino events with better angular reconstruction, resulting in an analysis that is three times more sensitive than the previous search,” says IceCube member, TU Dortmund physics PhD student, and co-lead analyzer Mirco Hünnefeld.
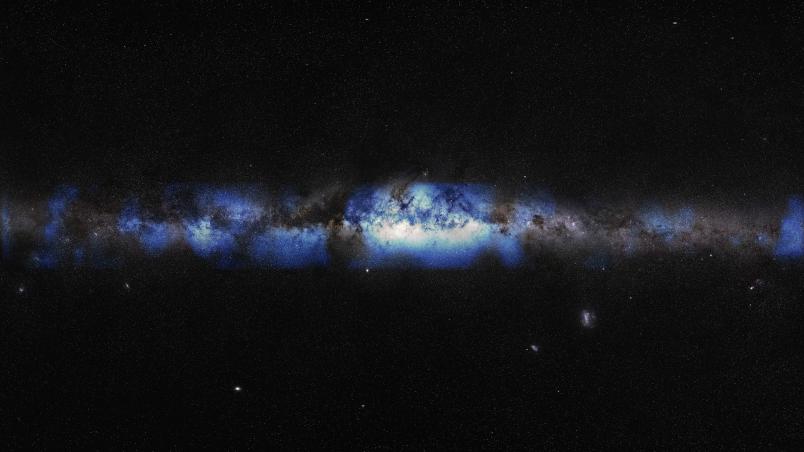
The dataset used in the study included 60,000 neutrinos spanning ten years of IceCube data, 30 times as many events as the selection used in a previous analysis of the galactic plane using cascade events. These neutrinos were compared to previously published prediction maps of locations in the sky where the galaxy was expected to shine in neutrinos.
The maps included one made from extrapolating Fermi Large Area Telescope gamma-ray observations of the Milky Way and two alternative maps identified as KRA-gamma by the group of theorists who produced them. “This long-awaited detection of cosmic ray-interactions in the galaxy is also a wonderful example of what can be achieved when modern methods of knowledge discovery in machine learning are consistently applied.” says Wolfgang Rhode, professor of physics at TU Dortmund University, IceCube member, and Hünnefeld’s advisor.
The power of machine learning offers great future potential, bringing other observations closer within reach. “The strong evidence for the Milky Way as a source of high-energy neutrinos has survived rigorous tests by the collaboration,” says Ignacio Taboada, a professor of physics at the Georgia Institute of Technology and IceCube spokesperson. “Now the next step is to identify specific sources within the galaxy.”
These and other questions will be addressed in planned follow-up analyses by IceCube. “Observing our own galaxy for the first time using particles instead of light is a huge step,” says Naoko Kurahashi Neilson, professor of physics at Drexel University, IceCube member, and Sclafani’s advisor. “As neutrino astronomy evolves, we will get a new lens with which to observe the universe.”
The Ruhr region in the IceCube consortium
Since 2009, Ruhr University Bochum has been part of the IceCube collaboration headed by Professor Julia Tjus, in close partnership with TU Dortmund University. In addition, Professor Dr. Anna Franckowiak and her chair of Multiwavelength Astronomy have been a member of the IceCube team since 2020. The researchers work primarily on the search for neutrino sources and continue to refine the IceCube software, which is used in the search for astrophysical neutrinos. The Bochum-Dortmund team is focusing specifically on analysis strategies with which astrophysical neutrinos can best be made visible. The crucial factor here is the combination of Bochum’s expertise on the sound theoretical background of neutrino emission with Dortmund’s research focus on advanced analysis methods based on artificial intelligence. As part of the Collaborative Research Centre 1491, Ruhr University Bochum is collaborating with TU Dortmund University on particle emission from the galactic disc. “The results obtained in Dortmund are an ideal starting point for us here in Bochum to continue working on understanding neutrino emission from the Milky Way,” comments Julia Tjus.