Newsportal - Ruhr-Universität Bochum
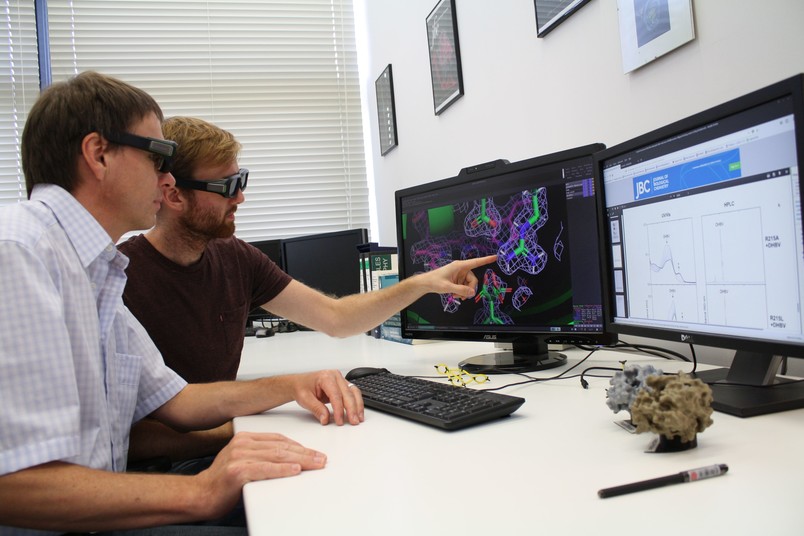
How algae produce pink pigments
Algae are capable of producing pigments in different colours from one and the same precursor molecule – depending on what they require in their respective environment. A team from the Faculty of Biology and Biotechnology at Ruhr-Universität Bochum (RUB) and Technische Universität Kaiserslautern analysed the biosynthesis of the pink pigment phycoerythrobilin in detail. The researchers discovered that a key enzyme permits a substrate to bond in only one unexpected orientation, thus generating the respective colour. The research team published its report in the Journal of Biological Chemistry on 20 September 2019. Their findings were featured on the cover of that edition.
Structurally very similar enzymes can generate pigments of different colours
The variety of colours in algae is the result of natural pigment molecules that are produced by the organisms in accordance with their respective environmental requirements. They are essential for algae to perform photosynthesis. Cyanobacteria, red algae and so-called cryptophytes use bilins for that purpose. Unlike the familiar green pigment chlorophyll, bilins are pigment molecules with a broad range of colours. Biliverdin is produced by enzymatic cutting of the ring structure of heme and serves as a precursor for all other bilins.
In the next steps, electrons and protons are specifically deposited at different positions in the intermediates. “The fascinating thing is that structurally very similar enzymes, known as bilin reductases, can generate pigments of different colours,” says Johannes Sommerkamp, member of Professor Eckhard Hofmann’s research group at RUB. “We do not yet fully understand the mechanisms controlling these chemical reactions.”
Cryptophytes are unicellular eukaryotic algae that are capable of photosynthesis and constitute a major percentage of the phytoplankton in the ocean.
Johannes Sommerkamp has studied specifically the synthesis of the pink phycoerythrobilin. “Phycoerythrobilin in algae is typically synthesised in two steps by two related enzymes. We successfully identified the structure of the enzyme for the first step several years ago, but we could not understand why the enzyme for the second step is so specific,” explains Eckhard Hofmann.
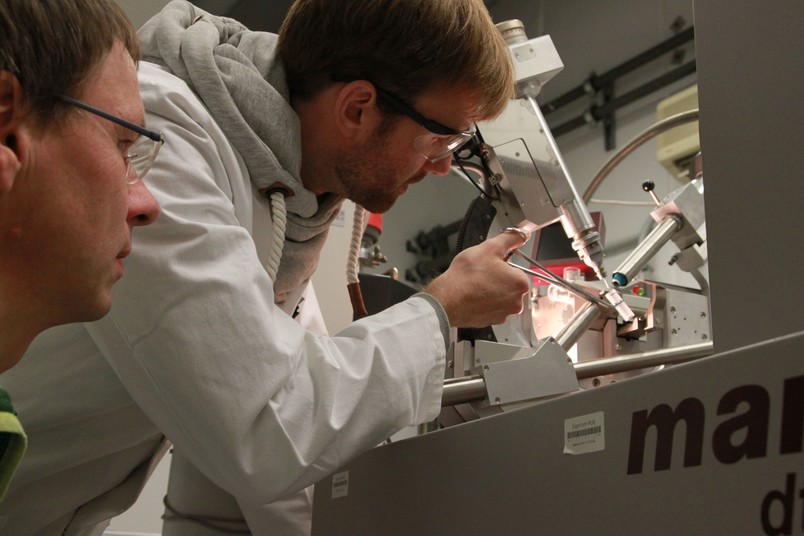
Johannes Sommerkamp has now decoded the three-dimensional spatial structure of the second enzyme from the cryptophyte Guillardia theta. He deployed X-ray structure analysis for this purpose, in which crystals of the enzyme are first grown and then examined with X-rays. Based on the collated data, a model of the enzyme with atomic resolution can subsequently be created. In the process, the researchers detected an unexpected orientation of the precursor pigment in the binding pocket and a catalytically relevant amino acid that plays a central role in the control of reactivity.
“If we examine the spatial structure of the binding pocket, it emerges that the substrate fits only in this orientation. This also determines how the reaction takes place,” points out Eckhard Hofmann.
The research is part of a joint project conducted with Prof. Dr. Nicole Frankenberg-Dinkel from Technische Universität Kaiserslautern and funded by the German Research Foundation. Both groups had previously jointly investigated a protein that combines the reactivity of both enzymes in one.
Johannes A. Sommerkamp, Nicole Frankenberg-Dinkel, Eckhard Hofmann: Crystal structure of the first eukaryotic bilin reductase GtPEBB reveals a flipped binding mode of dihydrobiliverdin. In: Journal of Biological Chemistry, 2019, DOI: 10.1074/jbc.RA119.009306
Prof. Dr. Eckhard Hofmann
Protein Crystallography Group
Faculty of Biology and Biotechnology
Ruhr-Universität Bochum
Phone: +49 234 32 24463
Email: eckhard.hofmann@rub.de
Prof. Dr. Nicole Frankenberg-Dinkel
Technische Universität Kaiserslautern
Department of Biology, Division of Microbiology
Phone: + 49 631 205 2353
Email: nfranken@bio.uni-kl.de
26 September 2019
10.40 AM